Introducing Symmetric Cells
Latest updated: October 24, 2023Introduction
Cells with identical electrodes, or symmetric cells, are used to study the behavior of battery electrodes [1], to study insertion reactions [2] and for determining the impedance of positive and negative electrodes in batteries [3]. They are also used to study Li battery electrodes [4].
Symmetric cells are composed of two identical electrodes where redox reactions occur, separated by a liquid or solid ionic conductor where charges are carried by ionic transport. The main advantage of using symmetric cells is to have two similar interfaces under study, whereas in full cells or even in half-cells two different interfaces are involved.
In batteries, electrodes are complex compounds composed of many different chemicals: active materials, binders, electronic conductors and so on…
Symmetric cells allow us to understand the interaction and electrochemical stability of each of these compounds under oxidation and reduction with a given electrolyte or a given electrode.
Here we will consider the case where the interaction of an electrolyte with metallic Li is studied, and more precisely its electrochemical stability.
Electrochemical reactions
Let us consider a symmetric cell composed of two Li electrodes separated by an electrolyte containing the Li$^+$ cation. The voltage difference across the cell is 0.
If a positive current is applied, ($I>0$) one of the electrodes becomes an anode and the following oxidation reaction will occur at its interface:
$$\text{Li} \rightarrow \text{Li}^+ + \text e^- \tag{1}$$
The other electrode becomes a cathode and the following reduction reaction occurs at its interface:
$$\text{Li}^+ + \text e^- \rightarrow \text{Li}\tag{2}$$
If a negative current is applied ($I<0$), the roles of each electrode are inverted: the former cathode becomes an anode and the former anode becomes a cathode.
Response to current steps
Figure 1 shows the potential response of a symmetric Li cell (Figure 1b) submitted to positive and negative current steps (Figure 1a). According to Bard and Faulkner [5], $\tau_1$ is named transition time constant beyond which “the flux of О to the electrode surface (in the case of a reduction reaction) […] is not large enough to satisfy the applied current, and the potential shifts to a more extreme value where another electrode process can occur”.
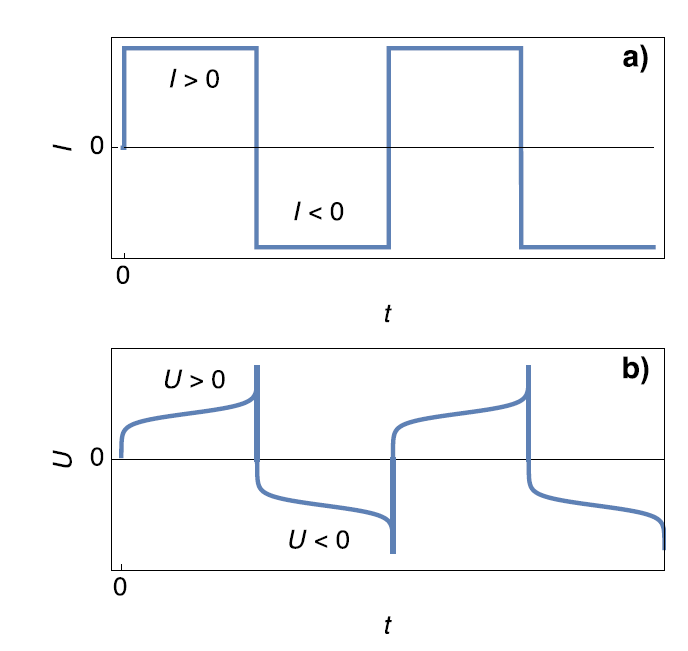
Figure 1: Scheme of a) galvanostatic current steps and b) voltage response for a symmetric Li-Li cell. $U=E_1-E_2$.
In the case of $I>0$, the rapid potential increase at $t=\tau_1$ can correspond to the complete consumption of the Li electrode. This is illustrated by Figure 2.
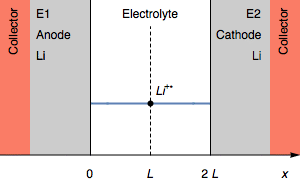
Figure 2: Evolution of the concentration profile and of the electrodes of a symmetric cell for a transition due to total anode oxidation.
In Figure 2, The thickness of the Li anode is 0 at the transition time. The further oxidation process could be the oxidation of the current collector. In the initial condition, $E_1=E_2$ and then $E_1>E_2$. We also have $E_1-E_2=U$, shown in Figure 1.
Still, in the case where $I>0$, the rapid potential increase can also correspond a complete depletion of Li$^+$ $( C_{\text{Li}^+} = 0)$ at the electrolyte|Li interface on the cathode. This is illustrated by Figure 3.
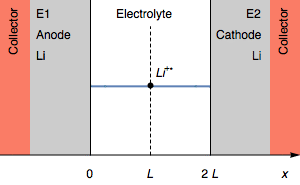
Figure 3: Evolution of the concentration profile and of electrodes of a symmetric for a transition due to interfacial cation depletion Li$^+$.
The interfacial Li$^+$ concentration on the cathode drops to 0. The further reduction process can be the reduction of the solvent. In the initial condition, $E_1=E_2$ and then $E_1>E_2$. We also have $E_1-E_2=U$, shown in Figure 1.
In the case of $I<0$, the same phenomena are also at stake but the role of the electrodes is switched. In the initial condition, $E_1=E_2$ and then $E_1<E_2$, electrode 2 becomes an anode and electrode 1 becomes a cathode.
Electrode stability
Symmetric cells are a simple and empirical way to test the stability of electrodes during galvanostatic cycling. In the case of Li, oxidation can lead to surface degradation and metal deposition (Li$^+$ reduction) can lead to dendrite formation. Figure 4a shows the voltage response of a stable cell and stable electrodes, where the amplitudes of the voltage do not change with time, and Figure 4b shows the voltage response of an unstable cell, composed of unstable electrodes, where the potential amplitudes do change with time.
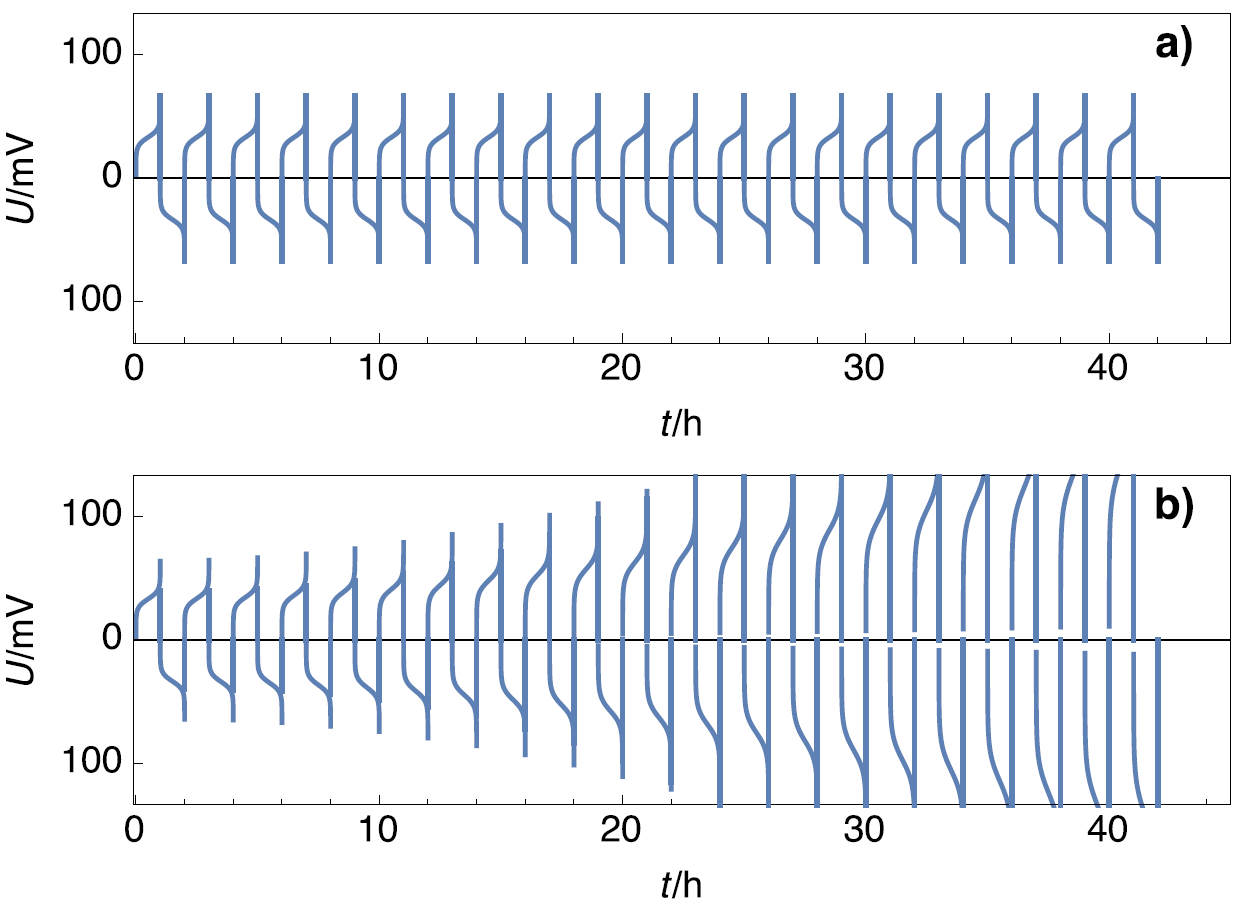
Figure 4: Scheme of potential profiles for a) stable electrodes and b) unstable electrode.
Transition times
Total oxidation of the Li anode
Assuming that the oxidation of the anode occurs via the process shown in Eq. (1), the transition time $\tau$ only depends on the mass of the electrode $m_{\text{Li}}$, which defines the charge necessary to fully oxidize the anode, and the applied current. If we define the charge and the current by unit area, $Q$ and $i$, respectively, we have:
$$\tau=Q/i \tag{3}$$
Replacing the quantity of electrons $Q$ per unit area, with a quantity of Li per unit area, we have:
$$\tau=\frac{m_\text{Li}F}{M_\text{Li}i} \tag{4}$$
Where $M_\text{Li}$ is the atomic mass of Li $(M_\text{Li}=6.94\,\text g\cdot\text {mol}^{-1})$ and $F$ the Faraday constant.
Interfacial depletion of Li$^+$ at the electrolyte|Li interface
Assuming that the reduction of Li$^+$ occurs via the process shown in Eq. (2), the transition time $\tau$ can be now calculated by the Sand equation [5]:
$$\sqrt{\tau}=\frac{FC_{\text{Li}^+}^*\sqrt{\pi D_{\text{Li}^+}}}{2i} \tag{5}$$
With $C_{\text{Li}^+}^*$ and $D_{\text{Li}^+}$, the bulk concentration and the diffusion coefficient, respectively, of Li$^+$ in the electrolyte.
Knowing how the current density affects the transition time allows us to better understand which mechanism is responsible for the potential increase/decrease defining the time transition.
Example
Figure 5 shows the evolution of the voltage as a function of time for galvanostatic current density steps of opposite sign, performed at three different values, on a symmetric Li|Li cell.
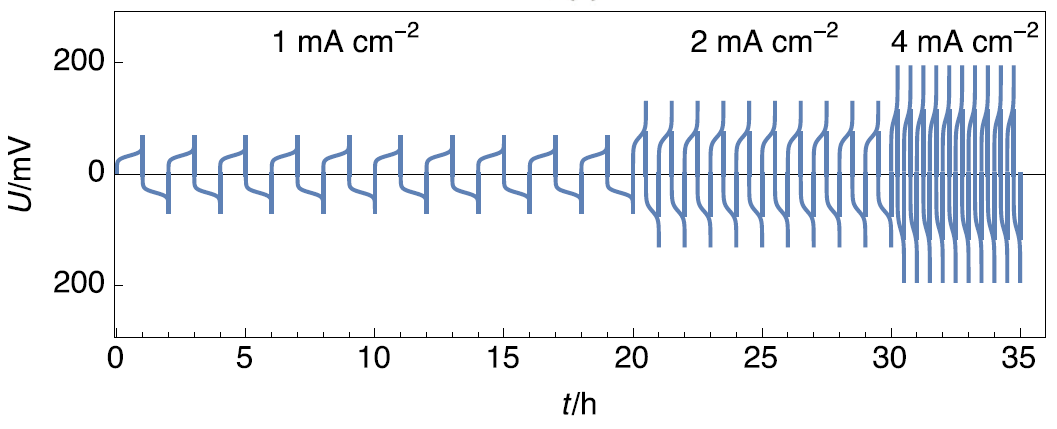
Figure 5: Chronopotentiometry performed with increasing current densities, adapted from Figure 3 in [6].
It can be seen that when the current density is multiplied by 2, the transition time in both current directions is divided by 2. This shows that Eqs. (3-4) can be used to describe the transition time dependency with time and that the controlling mechanism is the total oxidation of Li.
Conclusion
Symmetric cells can be used effectively to study and test the stability of Li electrodes in contact with solid electrolytes and the ability of these electrolytes to prevent the formation of dendrites. By using two identical electrodes, one can easily study the anodic and cathodic behavior over time and cycle number of one single electrode|electrolyte interface, without the need of a reference electrode. However, to go further into degradation mechanisms studies a three electrode configuration is recommended [7].
References
[1] C.H Chen, J. Liu, and K. Amine. Symmetric cell approach towards simplified study of cathode and anode behavior in lithium ion batteries. Electrochemistry communications, 3(1):44-47, 2001.
[2] J. C. Burns, L. J. Krause, Dinh-Ba Le, L. D. Jensen, A. J. Smith, D. Xiong, and J. R. Dahn. Introducing symmetric Li-ion cells as a tool to study cell degradation mechanisms. Journal of The Electrochemical Society, 158(12):A1417, 2011.
[3] J. Conder, C. Villevieille, S. Trabesinger, P. Novák, L. Gubler and R. Bouchet. Electrochemical impedance spectroscopy of a Li-S battery: Part 1. Influence of the electrode and electrolyte compositions on the impedance of symmetric cells. Electrochimica Acta, 244:61-68, 2017.
[4] Shang-Sen Chi, Yongchang Liu, Wei-Li Song, Li-Zhen Fan, and Qiang Zhang. Prestoring lithium into stable 3d nickel foam host as dendrite-free lithium metal anode. Advanced Functional Materials, 27(24):1700348, 2017.
[5] A. J. Bard and L. R. Faulkner. Electrochemical methods. Fundamentals and Applications. John Willey & Sons, New York, p. 308, 1980
[6] H. Wang, P. Hu, X. Liu, Y. Shen, L. Yuan, Z. Li, and Y. Huang. Sowing silver seeds within patterned ditches for dendrite-free lithium metal batteries. Advanced Science, 8(14):2100684, 2021.
[7] Battery cycling with reference electrodes using the PAT-cell test cell
Added 10/2023
Related products
Related accessories